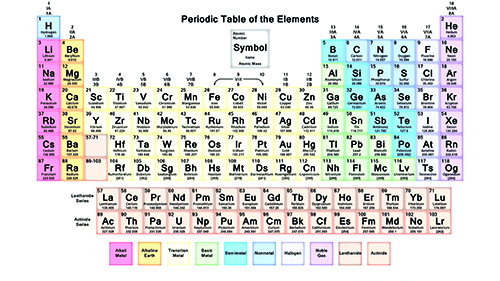
By Mark Leatherman
Gold, silver, and platinum. The big three of the precious metals realm. They are rare, beautiful, expensive, and typically smaller-sized specimens are represented in most collections. Most collectors are only able to be awestruck and take pictures of such large cabinet pieces that high-end collectors can possess.
Whether it is for investment purposes or having something akin to owning a Rembrandt or Picasso, it is no secret that precious metals are highly sought after. Despite their beauty and high value, it seems like the true scientific value of the precious metals tends to be overlooked. With that, we shall dive into the true origins of these prized metals, and how their placement on the Periodic Table not only makes them precious, but rather unusual!
Exploring Nucleosynthesis
As with any other chemical element, the precious metals were born billions of years ago when our galaxy began to take shape with the Big Bang. Element nuclei are synthesized in the center of stars at temperatures of millions of degrees and a level of pressure that makes forming diamonds in laboratories sound like a cakewalk; a process known as stellar nucleosynthesis. Atoms of hydrogen and helium, the simplest two elements by atomic number and what comprises 99% of the universe, are fused to build bigger nuclei of increasing atomic number.
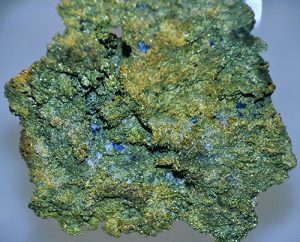
This process within stars continues until the iron is synthesized (atomic number 26), wherein iron nuclei are so tightly bound that fusion cannot continue at existing temperatures and pressures. Secondly, the fusion of iron atoms does not produce additional energy, as a byproduct, as lighter elements do. Without a continuous energy (or fuel) source, a star’s days become numbered where a supernova will soon follow.
During a supernova event, the star’s core collapses on itself then explodes, expelling all its created elements out into space. The core collapse event takes place in fractions of a second, and for that time, the sharp temperature and pressure increases are sufficient to fuse the heavier nuclei before expulsion. If we take a quick look at the Periodic Table, with most of the elements below atomic number 26, we can relate them to how common they are on and above the Earth’s surface. It is no coincidence that the eight most common elements in the Earth’s crust (O, Si, Al, Fe, Mg, Na, K, Ca) fit this pattern. Needless to say, the greatest amount of hell was raised to give birth to the precious metal beauties!
Another intriguing pattern seen in the elements is the fact that those with an even atomic number are generally more abundant in the universe, this being known as the Oddo-Harkins Effect. This can also be explained with stellar nucleosynthesis and, once again, with hydrogen and helium. The typical progression that happens in stars is that two hydrogen atoms (with one proton each) fuse to form helium, then two helium atoms bond to form beryllium, with the process keeping on forming nuclei with even-numbered protons every time.
With this, one may wonder how odd-numbered nuclei come about (including silver and gold with atomic numbers of 47 and 79, respectively). The most straightforward solution comes in the form of a nuclear process called neutron capture.
Within a Supernova
During a supernova explosion, a massive quantity of neutrons from the star’s core are shot out (like pellets from a buckshot shotgun shell) and incorporate themselves into the newly-formed heavier nuclei also shot out.
As more and more neutrons are added to a single nucleus, it eventually becomes unstable and undergoes another nuclear process known as beta decay. It is important to note that it is the protons that dictate an atom’s elemental identity, not the neutrons (the former serves as an atomic fingerprint of sorts.
With beta decay, a neutron turns into a proton and electron, and it’s this mechanism that adds one proton to the nucleus. Hence the atomic number goes up by only one to give the odd-numbered elements. Additionally, odd-numbered elements can also be achieved by trace amounts of unfused, “residual” hydrogen atoms being fused with any of the heavier nuclei as well.
At the time of its “birth” roughly 4.6 billion years ago, our planet was
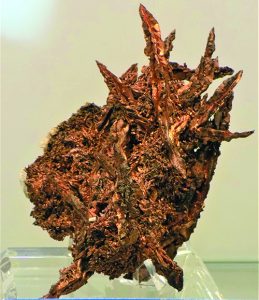
thought to be a very hot homogeneous mixture that has separated into density-stratified layers (much like what oil and water do). Elements such as iron, manganese, cobalt, nickel, molybdenum, gold and the platinum group (e.g. Pt, Pd, Ru, Rh, Re, Ir, and Os) are called siderophile (iron-loving) elements, in which they have sunk into the core, out of our mineable reach.
In this case, silver has fared a little better being classified as a chalcophile (copper-loving) element, along with the likes of sulfur, lead, zinc, and tin, to name a few.
Mineable State of Precious Metals
These elements are concentrated mainly in the upper mantle, which can then be brought to mineable levels via tectonic uplifting, magmatic, and hydrothermal fluid activity. With this, the only reason that we can access gold and the platinum group elements (PGEs) is due to an astronomical stroke of luck. It is thought that around four billion years ago, a swarm of meteorites impacted the Earth in an event known as the Late Heavy Bombardment.
The theory is the most likely explanation of how we can get mineable quantities of precious siderophile elements at crustal levels. A modern-day connection to this is the observation of a $5.4 trillion meteorite containing approximately 90 million tons of platinum passing near Earth in the summer of 2015 (and prompting the attention of prospective “space miners”). The platinum is alloyed with iron and nickel, all being chalcophile elements. Imagine how society might be different if the bombardment did not happen, and silver ended up being the chief of all precious metals rather than playing second fiddle!
With their violent histories established, we can take an even closer look within the precious metal atoms for more information about how they tick. General chemistry tells us two things: 1) that with a few exceptions, elements that appear in the same column (also called “groups”) tend to have similar properties, and 2) within an atom, it is the electrons that govern most of an element’s properties such as reflectiveness, propensity for oxidation, and color. Although all electrons of an atom are important for chemical properties, it Is the outer (valence) electrons that are the workhorses here. With that, the expression that denotes the distribution of electrons within the atom is known as the electron configuration.
To the chemistry tyro, the configuration for silver is seemingly [Kr]5s24d9, whereas gold’s configuration appears to be [Xe]6s24f145d9. The larger numbers (called coefficients) indicate electron energy levels or relative distance from the nucleus. The letters indicate the geometric-shaped “cloud” (or orbitals) where the electrons probably reside (they are always on the move). The smaller numbers (or superscripts) are the number of electrons (in a neutral atom, the superscripts add up to the atomic number). As you can see in silver and gold, they both have 9 “d” electrons (a “d” shell can hold a maximum of 10 electrons, just count the number of Periodic Table squares across any complete row of transition elements), and it is in the d shell where some interesting phenomena takes place!
For both of those metals, they are only one electron away from having a full “d” shell. Additionally, electrons love to work in pairs, and atoms seem to prefer orbitals that are either full, half-full, or empty. What happens with silver and gold is that one electron from the “s” orbital is transferred to the “d” orbital, to make it full and leave the “s” orbital half full. Thus, the respective true electron configurations are [Kr]5s14d10, whereas gold’s configuration is [Xe]6s14f145d10. It is the movement of the one lone “s” electron that gives rise to the highly illustrious nature and very high electrical conductivities. Silver is the most reflective element, reflecting 99% of the light that hits it, whereas gold reflects around 95%. Oftentimes, high shimmer and preciousness go together, and we have a single electron with nearly no mass to thank!
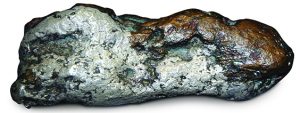
Energy, Wavelengths and Color
It is no secret that the luster and color of gold have made it the metal of envy for millennia, but what exactly is it that gives gold that famous yellow hue? Introductory chemistry students are often introduced to a two-dimensional Bohr Model of the atom to explain not only the number of valence electrons an element has but also how electrons jump from “ring to ring”, or between energy levels when they absorb energy. Visible, or white, light contains a fixed range of energy as denoted by the electromagnetic spectrum.
The electrons absorb the energy that allows them to “jump” to higher energy levels. When said electrons return to their original energy level, they release a quantity of energy in the form of certain wavelengths associated with certain colors.
In the case of gold, it is the blue wavelengths that are absorbed with the complimentary yellow hues being reflected. If only that explanation were that simple! Along with jumping electrons, we can contribute gold’s “odd” color to quantum mechanics and Einstein! Along with Erwin Schrodinger in the mid-1920s, Einstein helped develop the more contemporary and accurate model of the atom, which is known as the quantum-mechanical model.
One of the primary differences between the two models is how the areas where electrons live are described. Electrons technically live in geometrical “clouds” of probability called orbitals. Like with the Bohr model, orbitals exist in increasing sizes or energy levels away from the nucleus.
Accompanying most heavier nuclei is a concept called relativistic effects, which was also envisioned by Einstein. It should also be noted that the following explanation of how these effects affect precious metals is very simplified.
All electrons zip around the outside of atoms, within orbitals, at extremely high speeds. Typically, an infusion of energy, such as heat, light, or electricity, will increase an electron’s movement or kinetic energy. In some of the heaviest nuclei, electrons travel at speeds comparable to that of light. Think of this as moving planetary bodies. Bigger bodies (heavier nuclei) possess a stronger gravity, which makes objects near it (electrons) move faster.
When this happens, an additional influx of outside energy will not increase the speed even more but rather add on to the atom’s mass in a phenomenon known as relativistic mass. In theory, all objects have relativistic mass, but mere mortals cannot detect it because we, and most other objects, move at such a “slow” speed. Thus, it is negligible.
The German physicist, Arnold Sommerfeld (1868-1951), deduced that an electron’s speed could be found by the equation v = Zc / 137 (where c is the speed of light also used in Einstein’s most famous formula E = mc2, and Z is the atomic number of the atom considered). In the case of a gold electron (where Z = 79, the highest atomic numbered considered here), it moves at around 58% the speed of light. This is considerable enough for relativistic effects to be significant; in gold’s case, it is an “abnormal” color.
Different Effects of Different Elements
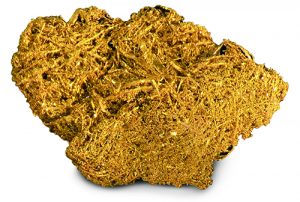
It should be noted that relativistic effects are an overarching, collective term indicative of different effects on different elements. Perhaps it is no coincidence that gold and mercury are right beside each other on the Periodic Table and compared to normal metals that both are “oddballs” due to relativity, with mercury’s effects being that it’s liquid. Relativistic effects have a second and very important effect on gold, without which we may never view gold with the same value despite its color. Due to its very full “d” electron orbital, housing fourteen electrons (and all at very high speeds, no less), it pulls in or contracts the one lonely 6s closer to the nucleus and shields it from being transferred (or bonded to another element) in a reaction. This contraction allows an electron to jump from the 5d to the 6s level at energy equating to the release of a yellow wavelength.
Without the contraction, the energy required for the same jump would be in the ultraviolet (non-visible) range. With the 6s valence electron now being “protected”, only the most reactive substances on Earth can yank it out. Hence, the electron shielding is attributed to gold’s non-reactiveness or inert state. When King Tut’s sarcophagus was unearthed nearly a century ago, the gold comprising it still had its shimmering brilliance from when it was first crafted many millennia ago.
In comparing silver to platinum, the latter experiences the same shielding effect as gold, also giving rise to platinum’s tarnish resistance. The same cannot be said for silver since it lacks the “f” electron orbitals to provide the shielding effect and is attacked over time by the likes of oxygen and sulfur. Despite this, silver is still considered a “noble metal” that takes some time to degrade. If one were to peek at a tool called the activity series, the precious metals are neatly gathered at the bottom of this “totem pole.” With this pole, however, being at the bottom is great. It signifies an unwillingness to give up electrons in reactions, which leads to oxidation. At least silver is nowhere near as bad as iron.
Copper’s Unique Contribution
In reviewing the fact that gold and silver have similar properties, by being in the same column or family, it is easy to spot that copper is in the same boat. However, in the precious metal realm, it is often snubbed due to its relatively common occurrence and of course, low cost. I often tend to think of copper as precious in terms of its contribution to early human civilizations with the Bronze Age. For the above two reasons, I will include the red metal here.
Copper’s electron configuration is [Ar] 4s13d10 and possesses nearly the same relativistic effects as gold but on a slightly smaller scale. Since copper is a smaller atom than gold (it possesses less filled energy levels), the electron jumps between the 4s and the 3d levels do not require as much energy. This is manifested as a lower energy red-orange wavelength being released versus the slightly higher energy orange-yellow wavelengths of gold thanks to its additional energy levels. Lastly, copper is the most prone to oxidation since it experiences the least amount of electron shielding, with relativistic contraction, simply due to its least number of electrons and energy levels (hence its higher status on the activity series). Although it is by far the most common metal considered here, it is priceless in terms of advancing early civilizations. Think of how behind we could be today if someone wasn’t intrigued by copper’s redness.
For anyone that has collected specimens of gold, copper, and silver, one has got to be struck by the myriad of wondrous crystal forms such as cubes, dendritic, wires, octahedral, sheet, herringbone, and spinel law twin to name several. Although all three crystallize in the cubic class, the various crystal forms of any mineral are a direct reflection of the environment they grew in; more specifically with pressure, temperature, pH of the crystallization medium, as well as salinity (i.e. other minerals dissolved with the precious metals). It is no coincidence that gold, silver, and copper display the same forms by being in the same family.
When it comes to the crystal forms of platinum, and the rest of the PGEs, it is a slightly different story. Although they also crystallize in the cubic system, they chiefly form in a more specialized environment. Whereas the other three metals can be found in a host of geological systems and host rocks, the PGEs preferably are found in mafic igneous rocks, with the clear majority of said rocks being part of layered mafic intrusions (LMIs) emplaced in shallow levels of the crust. The number of native copper, silver, and gold locales is far too numerous to list, but those of platinum can be displayed here (list).
The PGE preference for all things mafic comes from their proximity to iron on the Periodic Table, a dominant element in mafic rocks such as basalt, gabbro, and peridotite. Given this “one-dimensionality” of platinum, nearly all well-formed specimens are cubes that have formed under a restricted temperature and depth range.
Given the extremes required for their formation, their quantum eccentricities, and some “astronomical” luck, hopefully, after reading this you will have gained a much deeper appreciation for the Picassos of the native metal realm no matter what level collector you are, and no matter the size of such specimens in your collection.